Today, April 25, is World Malaria Day. This infectious disease is caused by unicellular parasites from the genus Plasmodium and spread by mosquitos. It affects hundreds of millions of people from across the world. In recognition of World Malaria Day, we spoke with Virgilio L. Lew and Teresa Tiffert from University of Cambridge about their research relating to malaria.
What is the connection between your research and malaria?
Our connection to malaria was prompted by many open-ended questions on the biology of Plasmodium falciparum infected human red blood cells which we thought could be answered using biophysical methods and mathematical models previously developed and applied in our laboratory in studies on the homeostasis of red blood cells in health and disease.
Why is your research important to those concerned about malaria?
When the malaria parasite P. falciparum, responsible for the most deadly form of the disease, invades a human erythrocyte, it initiates a 48-hour reproductive cycle ending with the host red cell bursting and releasing dozens of parasites ready to invade neighbouring erythrocytes in the circulation. During this period the parasite induces major changes in the homeostasis of the host red cell. Our research centred primarily on trying to explain the origin of these changes focussing on two apparently unrelated and puzzling results. The first concerned the parasite-induced vast increase in the permeability of the host red cell membrane, which, if projected to an uninfected cell would lead to rapid lysis. How then was premature lysis prevented in the infected cell? The second question concerned haemoglobin consumption. The parasite ingests and digests up to 80% of the host haemoglobin at a high metabolic cost, yet most of the amino acids produced are unselectively discarded as waste through the increased permeability pathway. Why is so much energy wasted in digesting excess haemoglobin? To approach the answers we used a mathematical model of the homeostasis of the infected host red cell, built as an extension of our previous erythrocyte models. The model provided an unexpected answer. Excess haemoglobin consumption by the malaria parasite was essential to reduce the colloidosmotic pressure within the host red cell to prevent its premature lysis, a surprising connection between the two seemingly unrelated puzzles. A number of different model predictions have since been experimentally validated in different laboratories.
How did you get into this area of research?
It was a natural progression from studying the physiology of red blood cells to applying that understanding to disease conditions, like anaemias or malaria infection, in which red cell behaviour is altered. On the initiative of my long-standing collaborator, Teresa Tiffert, we adapted our laboratory to open a dedicated area for malaria work. As new questions arose, we began using imaging and optical tools, working with Pietro Cicuta and his team in the Physics Department, Cambridge. Over the years, we benefitted greatly from collaborations with colleagues not only in Cambridge but also Oxford, London, New York, and Jerusalem.
Do you receive public funding for this work? If so, from what agency?
Our research on malaria was generously supported at different times by the Medical Research Council (MRC), The Wellcome Trust, the Biotechnology and Biological Sciences Research Council (BBSRC), the Engineering and Physical Sciences Research Council (EPSRC), the Cambridge Newton Trust and the University of Cambridge.
Have you had any surprise findings thus far?
I will briefly survey a few here.
One surprise was the appearance of a calcium permeability pathway in the infected cell late in the infection cycle. Strangely, this path was active only if the cell became metabolically depleted. Why would the parasite introduce such a pathway? One potential explanation could be that this adaptation evolved to keep the human host alive during infection. The risk of death in severe malaria comes from infected red cells clogging vessels in the brain. Metabolic deprivation of the infected cells in such vessels would lead to calcium loading and apoptotic cell lysis, reducing blockage thus mitigating human host mortality.
Another surprise arose from a chance observation in our lab, which led to the discovery that dehydrated red cells are much less susceptible to malaria invasion. Interestingly, there are several inherited haemolytic anaemias in which red cells are dehydrated, and these genetic anaemias are prevalent in parts of the world where malaria is endemic. These findings suggested that such genetic traits may be advantageous due to the protection from malaria conferred by red cell dehydration.
With our physics collaborators, we developed a fully automated, robotic imaging platform to detect and capture random egress-invasion events. Unexpected variations in parasite egress modalities suggested asynchronies in the confluent processes that control parasite release.
We used electron-probe X-ray microanalysis to provide for the first time comprehensive maps of the elemental distributions of sodium, potassium and iron in malaria-infected cells. The figure Teresa Tiffert and I are scrutinizing here is a sample from that study, revealing the high-sodium (green) condition within the host red cell surrounding a high-potassium (red) parasite, with iron (blue) signals from the hemozoin crystals within the parasite food vacuole.
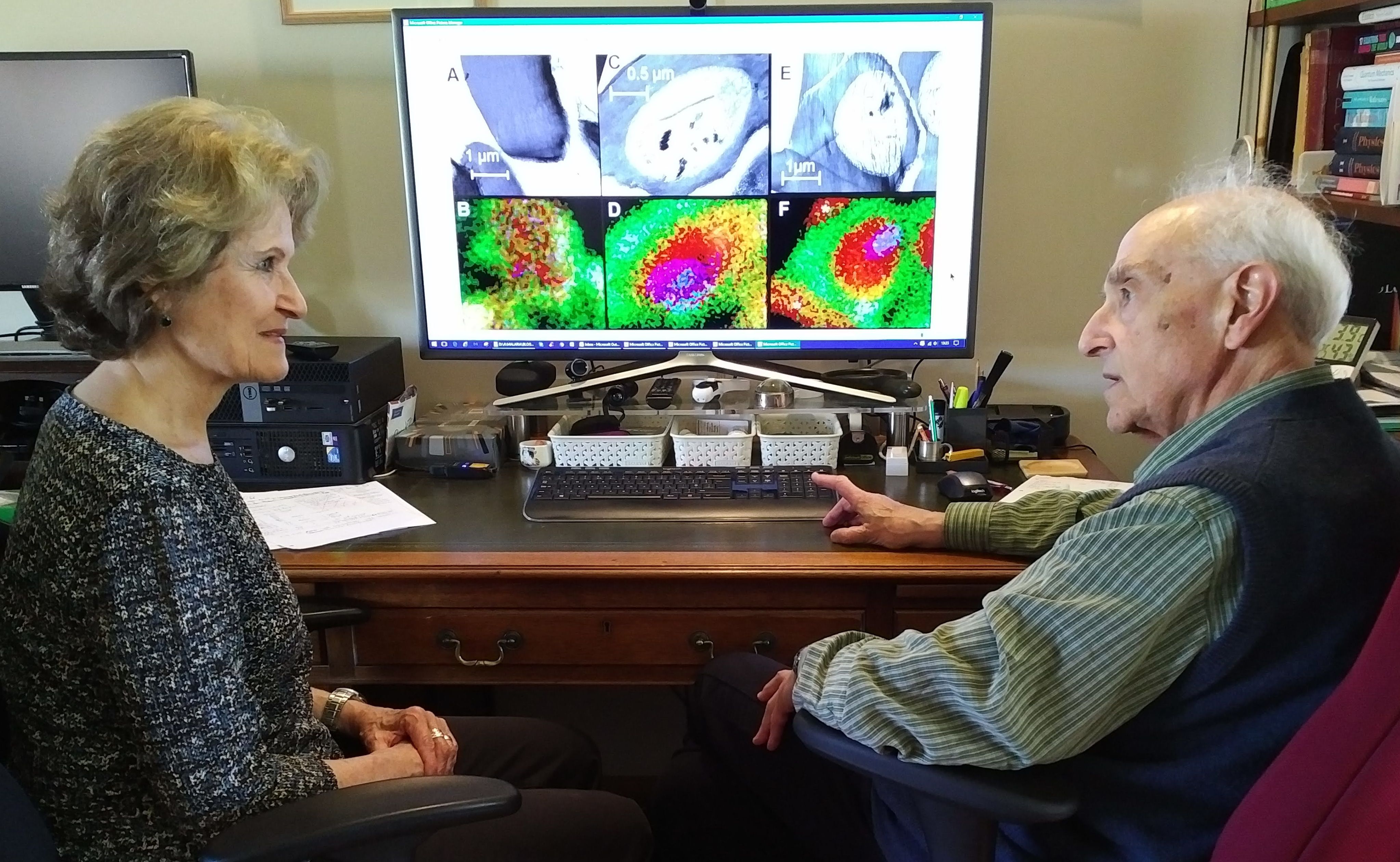
What is particularly interesting about the work from the perspective of other researchers?
Our model on the homeostasis of falciparum-infected red blood cell provides a reliable tool for researchers in the field to estimate the condition of the infected cell throughout the reproduction cycle of the parasite. Our work has stimulated an appreciation of the value of biophysical approaches to study host-parasite interactions in malaria.
What is particularly interesting about the work from the perspective of the public?
Basic research directed at answering fundamental issues on the biology of the malaria infection in the human host is often neglected by the media when there is no immediate therapeutic association. Because of this, many unanticipated instances of applications arising from original basic research findings have been overlooked. For example: while exploring whether a particular ion channel of human red cells became activated during malaria infection, we tested the effects of the widely used anti-fungal drug clotrimazole, which blocks that channel. We discovered that it had a powerful antimalarial effect, through a mechanism later elucidated by colleagues in Japan. This work has not been followed up yet with clinical trials, and clotrimazole remains part of a group of potential therapeutic agents to fall back on in a future of diminishing alternatives.