February is American Heart Month. Heart disease is the leading cause of death for men and women in the United States, causing 1 in 4 deaths each year. In recognition of this awareness month, we spoke with BPS member Anna Grosberg, University of California, Irvine, about her research on cardiomyocytes, which play an essential role in contracting the heart and pumping blood.
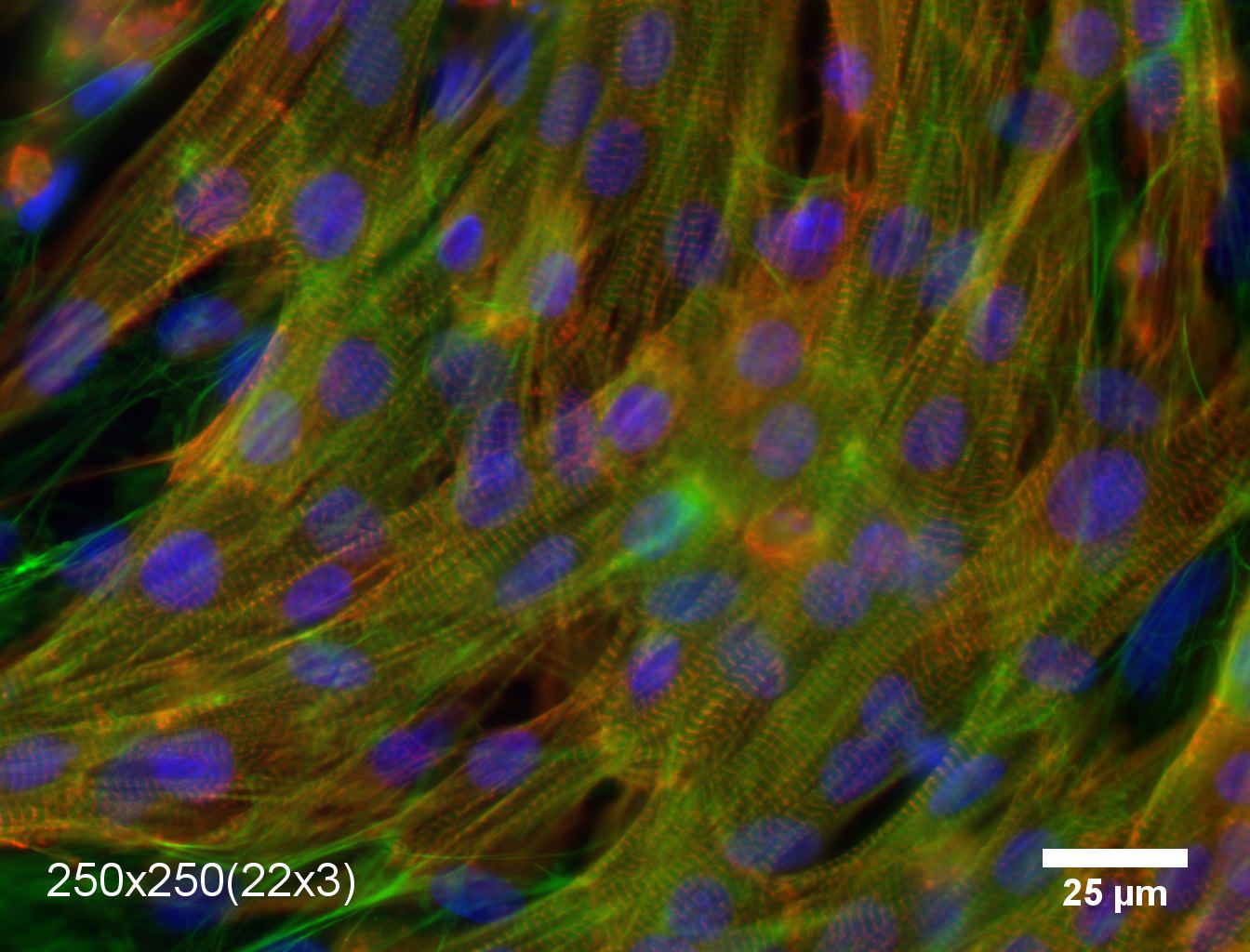
What is the connection between your research and heart disease?
My laboratory studies the structure dynamics, and function of cells. We are mostly focused on cells that make-up the heart muscle – cardiomyocytes, which play the essential role in contracting the heart and pumping the blood. Recently, we have made discoveries elucidating how the organization on multiple length-scales affects the ability of engineered heart tissues to generate force. This is especially important for the future progress in developing therapies following heart attacks and for understanding heart diseases, such as dilated and hypertrophic cardiomyopathies.
Why is your research important to those concerned about heart disease?
The label “heart disease” encompasses a wide variety of patient symptoms and pathologies. Yet in many presentations of the disease, the structure of the heart is changed over a wide variety of length-scales. Indeed, the normal heart consists of sheets of elongated cardiomyocytes arranged to generate maximal force in parallel with each other. Conversely after an infarct, a scar forms that disturbs this organization and prompts the muscle to remodel. Often such remodeling causes the cells to become less organized within the tissue, and it can also occur without an initiating scar. Understanding how these progressive architectural changes affect function is important to identifying the dominant mechanism to target in modulating heart disease. Through the type of research my lab does, it was discovered that changes to the organization also cause downstream variations to expression levels of a variety of genes some of which are linked to both electrophysiological and contractile function. This makes it problematic to determine how much of the force reduction of diseased muscle is caused by disorganization, and to what extent it is influence by the downstream biological effects. We have recently found that providing muscle cells with local organizational cues, while maintaining a global organization results in engineered cardiac muscle with predictable organization-force generation relationship based on simple physical understanding.
In general, remodeling of the heart muscle and, even, the enlargement of the heart are part of normal physiology. For example, it is known that for both competitive athletes and pregnant women, the heart can enlarge for the duration of the higher load, but it will remodel to normal size once the excessive exercises are over or the baby is born, respectively. Thus, there is hope that by understanding the functional aspects of remodeled heart tissue, it will be possible to reverse some of the pathological remodeling observed in heart disease. Additionally, we also work with cells from patients with genetic mutations that cause heart disease. A deeper understanding of the mechanisms that make these cells abnormal can provide more clues for how genetically normal patients can still develop heart disease.
How did you get into this area of research?
In the past decade, advances in tissue engineering have allowed researchers to guide the architecture of engineered heart tissues. It has also been possible to image the detailed structures within the tissues – thus visualizing the organization of the sarcomeres (the force producing units within muscle cells). However, it was also found that the reduction in force generated by badly organized tissues was two times higher than predicted from basic physics principles. Unfortunately, it was not possible to change the organization of the tissue without also triggering biological downstream effects that affected force generation in complex, poorly understood ways. When I started my lab, one of our big goals was to create a system to both quantify organization of cardiac muscle over multiple length-scales and to build an experimental system where the organization could be changed without triggering any downstream effects. These tools now allow us to greatly deepen our understanding of the relationship between structure and function in both normal and diseased heart tissues.
How long have you been working on it?
I have been working on the relationship between structure and function in the heart for the past 15 years. However, only in the past few years we have built-up the tools to study multi-scale organization and its connection to force generation.
Do you receive public funding for this work? If so, from what agency?
Yes, our work has been funded by both the National Science Foundation and the National Institutes of Health.
Have you had any surprise findings thus far?
We have found that in the absence of guidance cardiac tissue forms spontaneous patches of organization. Yet, the force generated by such a tissue is almost two times weaker than if the patches of organization are introduced with guided parquet patterns. The first surprising finding was that it is possible through very basic physical principles (i.e. force vector addition) to predict the amount of force generated tissues if their organization was guided in parquet tiles. Second, we found that the spontaneously organized patches are only 3 times smaller than the engineered parquet tiles. This implies that either the cells are very sensitive to a narrow range of local signals, or the temporal change in self-assembly provided by guidance cues plays an important role in resultant force generation. Our current aim is to solve this mystery.
What is particularly interesting about the work from the perspective of other researchers?
Our results have shown that in the case of eliminating biological downstream effects, simple physical principles are predictive for cardiac tissue force generation. This provides a way to interpret the reduction in force observed in stem-cell derived cardiomyocytes that are incapable of achieving sarcomeric structures that fully match the adult or even neonatal hearts.
What is particularly interesting about the work from the perspective of the public?
Heart disease is still the number one cause of death in the developed world. Yet, there are no cures – only therapies that help manage the disease. By exploring the basic physical principles behind heart muscle structure and function, it might be possible to provide better targets for therapies or surgical intervention. Additionally, it might become realistic to use the principles we discover to build better engineered cardiac tissues, which would provide better predictability for testing pharmaceuticals for cardiac toxicity.